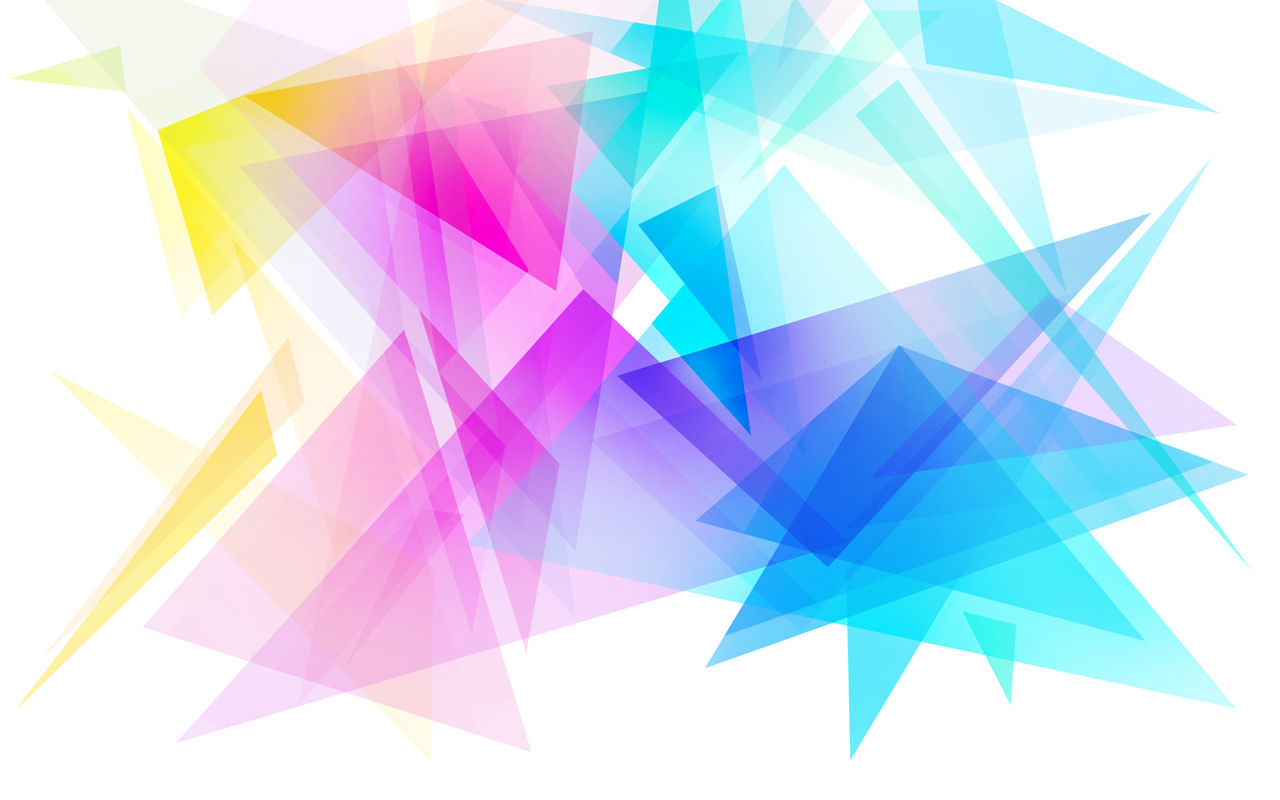
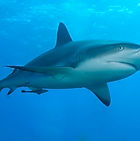
More efficient planes and boats
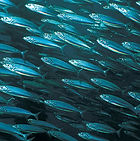
Robotic cars
A large proportion of all life on Earth exists in the oceans. Exactly how large the proportion is unknown, since many ocean species are still to be discovered. While the oceans constitute about 71% of the Earth's surface, due to their depth they encompass about 300 times the habitable volume of the terrestrial habitats on Earth.
The diversity of marine life is partly due to the vast array of habitats: from the tiny layers of surface water in which organisms may be trapped in surface tension between the ocean and atmosphere, to the depths of the oceanic trenches, sometimes 10,000 meters or more beneath the surface of the ocean, coral reefs, kelp forests, tidepools, muddy, sandy and rocky bottoms, and the open ocean zone, where solid objects are rare and the surface of the water is the only visible boundary.
Marine Life
Shoals of fish are able to move and turn in seamless formation by following simple rules that are like those used by car drivers, researchers say. Many theories have been put forward about how they might communicate in order to achieve this.
Images of swimming fish were fed into tracking software which acts like many pairs of eyes to keep tabs on the direction and speed of each fish in the school and how it responds to other fish around it. Because schools of fish rarely suffer from crashes or congestion, the rules they use could also potentially be applied to robotic systems to solve traffic problems.
If you could engineer a system where each car knew where each car was and they could respond to each other and communicate with each other, you would avoid traffic problems.
For millions of ships travelling through ocean waters, a process known as "bio-fouling" has serious implications. The collation of 'sea gunk' on ship hulls can increase a ship's drag by up to 15%. This reduces the ship's hydrodynamic efficiency, making it travel slower, consume more fuel and cause more pollution. Regardless of the speed at which sharks travel through water they don't pick up any forms of marine life on their skin. Mimicking the shark's scaly skin patterns on plastic has proved so successful that is has been adopted by the entire US navy fleet. The benefits of plastic 'shark skin' aren't just limited to ship and planes, the material was also used in the 2008 Olympic swimsuits, which were then banned for the advantage and the amount that they increased the swimmers' speed in the water.
In the case of the Mercedes Benz Bionic (2005) the boxfish was the inspiration for this environmental design. Unusually, the front two thirds of their bodies are encased in a bony structure that comes in all sorts of shapes—some triangular, others rectangular or even trapezoidal, ridges jut out over their eyes and bony keels stick outward from their bellies and backs.
In other words, it’s the last kind of fish one would expect to be aerodynamically smooth. However, looks can be deceiving and researchers found that the boxfish is actually and exceptionally stable and smooth swimmer. One other striking feature of the boxfish is how smooth it can swim. Its body had a lower than expected drag coefficient, which is a technical way of saying that it can move through the water with little resistance from the water itself. This was then adapted in the design of the car in order to reduce the drag and increase efficiency.
Tiny snails sitting on the ocean floor might seem defenceless against a large, determined predator such as a crab. But evolution has provided one species of sea snail with a unique iron-plated armoured shell that resists such attacks. The snail has evolved a tri-layered shell structure consisting of an outer layer embedded with iron sulphide granules, a thick organic middle layer, and a calcified inner layer.
By copying aspects of that shell structure, scientists could design better armour for military use. Such fundamental knowledge holds great potential for the development of improved biologically inspired structural materials, for example soldier, first-responder and military vehicle armour applications,” said Ortiz.
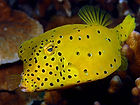
Eco-cars
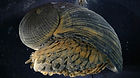
Armour
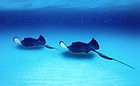
Efficient Robotics

Armour
Engineers at the University of Virginia are designing and building a bio-inspired robot that's based on stingrays and manta rays. Rays are wonderful examples of optimal engineering by nature, they're graceful swimmers, and can conserve energy by gliding for long distances.
The robot can swim, turn, accelerate and glide with a near-silent flap of its wing-like pectoral fins. Researchers control the vehicle remotely by computer commands. The aim is to achieve optimal silent propulsion with a minimum input of energy.
The ray bot has a few potential applications. It could be used to collect undersea data for scientists, or as a surveillance tool for the military. If scaled up (or down), it could even be a platform to carry payloads like monitoring instruments.
A massive Brazilian fish that can survive in piranha-infested lakes might have a few tips for engineers who are making flexible, impenetrable body armour.
Piranhas use their famous razor-like teeth to trap skin and muscle in a guillotine-like bite, and then tear the prey into bits. But the Arapaima sports intricately-designed scales that are tough enough to repel attacks, and even crack a predator's tooth.
The scales overlap each other like shingles, and each scale has a heavily mineralised outer layer like the hard enamel of a human tooth. The surface is corrugated, too, to allow it to bend without cracking. Underneath, softer collagen fibers are stacked in alternating directions like a pile of plywood. By combining a hard outer layer and a softer internal architecture, the scales are able to flex, bend and deform while remaining strong. This lets the fish remain mobile while staying heavily armoured, and alive.
The next step is to take the lessons learned from the Arapaima's scales and apply it to body armour. It could also improve fuel cells, insulation and aerospace design.
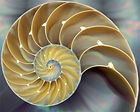
More efficient hydroelectric power

Self sharpening tools
Sea urchins have five teeth at the centre of their bodies. These teeth are able to chew through solid rock, making a cavity in which the sea urchin hides and withstands the surge of water currents. Scientists have long wondered how sea urchin teeth can withstand grinding and scraping against rock surfaces despite only being made of calcium carbonate (limestone or chalk) which is only moderately hard.
The calcite of its teeth is in the form of a cemented mosaic of plates and fibres. This composite structure greatly increases tooth durability. Between the plates is weaker organic material which functions as the ‘weak link’. It is at these boundaries where the tooth breaks. The tooth continues to grow equal to the loss due to breakage. And each fracture occurs in an angular way which keeps the edge of the tooth sharp. The renewable urchin teeth suggest application in industry: Self-sharpening blades for tools. On the scale of everyday tools, an analogy could consist of a slow-moving metal rod with a variable composition. On the scale of nanotechnology, cutting tools may be possible which continually grow and sharpen themselves.
The natural Fibonacci spiral shape, seen in the nautilus shell, has long been a favourite repeating pattern of nature and mathematics. Now employed as the Lilly Impellor a fan/propeller which requires 50% less energy, 75% quieter: therefore it reduces turbulence and reduces excess heat with half motor for same size fan. This could lead to hydroelectric dams without so much fish damage, or reduce the amount of space needed for computer fans, which will then reduced energy costs
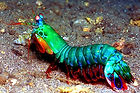
Dodechromatic DVDs
The eyes of a tiny marine crustacean may lead to the next generation of DVD and CD players. The mantis shrimp that inhabit Australia's Great Barrier Reef have the most complex vision system yet known to science. While humans see three colors (trichromatic vision), these shrimp see twelve, and can distinguish between different polarizations and even convert linear polarization to circular polarization and visa versa. No synthetic design can compete with the shrimp's natural methodology, comprised of cell membranes rolled into tubes. Better optical devices could be developed using liquid crystals chemically engineered to mimic this simple and effective crustacean technology.
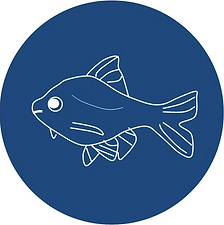
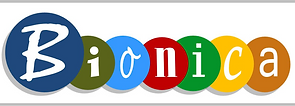